
Changed Aquatic Environment Due to An Estuary Dam
As a case study of the changed aquatic environment due to an estuary dam, the Geum River Estuary Dam System (GREDS) has been investigated for the last two decades. We sought the similarities and differences in the disrupted aquatic environment between the estuarine and lacustrine sides of the GREDS. Both sides of the GREDS shared similar aquatic disruptions, such as elongated hydraulic residence times, deteriorated water quality, highly accumulated organic-rich sediments, and considerable siltation of river-transported materials prior to reaching coastal waters. The disruptions of water quality such as high nutrients concentration and frequent bloom of blue-green algal are much more noticeable in the reservoir than in the estuary. However serious siltation problem has been reported from the estuary, which will possibly threaten the proper functioning of the natural Kunsan Estuarine System.
초록
금강 하구언 건설 이후 20년 동안 나타난 수서환경의 각종 변화에 관한 연구결과를 호수측과 하구측을 비교하는 방식으로 정리하였다. 유사한 점은 양측 모두에서 물의 체류시간 증가, 수질악화, 퇴적물내 유기물농도 증가, 하천유입 퇴적물의 이동경로 차단현상이 나타났다. 전체적으로 호수측이 하구측보다 악영향이 강하게 나타났지만, 호수측에서는 영양염농도의 증가와 남조류 대량발생 등 수질악화현상이 두드러지게 나타난 반면, 하구측에서는 퇴적물의 이동경로차단으로 인하여 군산하구내 퇴적현상이 심화되어 정상적인 하구의 기능을 저해하고 있다.
Keywords:
Aquatic Evironment, Estuary Dam, Water Quality, Siltation, Hydraulic Residence Time, 물환경, 하구댐, 수질, 토사퇴적, 수문학적 체류시간1. INTRODUCTION
Globally, almost 800,000 artificial lakes and reservoirs have been built to provide services such as drinking water supplies, hydropower production, irrigation, and flood control (McCully [1996]; Gleick [1999]). Most of them are inland dams, yet some are located at the river mouth, so called the estuary dams. More than 202 estuary dams have been built in Korea (KRC [2009]) and more in Japan (Sawaragi [1988]) for the last two decades. Many more estuary dams are scheduled to build in India, South-East Asia, and in other developing countries.
Since the pioneering studies on the environmental impacts of inland reservoirs and impoundments (Baxter [1977]; Rosenberg et al. [1997]), diverse investigations have been executed related to residence time (Rueda et al. [2006]), the Asian monsoon period (An & Jones [2000]; An & Park [2002]), suspended solids (Weyhenmeyer et al. [1997]; Jones & Knowlton [2005]), and phytoplankton (Søballe & Kimmel [1987]). Recently some remedies to mitigate these impacts have been suggested by artificial aeration (Tsujimura [2004]), siltation control (Håkanson [2004]; Förstner [2004]), and re-filling water (Lourantou et al. [2007]; Gelaldes & Boavida [1999]).
Estuaries, in nature, represent † a pathway for materials from land to coastal waters and function as a filtering system. This system includes the dilution of fresh water with salt water, the uptake and release of nutrients by living organisms, and the adsorption and release of metals by particulate materials. However, the artificial structures like the estuary dams disrupt their normal functions as estuaries. As one of such dams, the Korean Rural Community Corporation (KRC) constructed the Geum River Estuary Dam system (GREDS) across the Geum River mouth (Fig. 1).
Since the dam was built, the environmental impacts due to the GREDS have been extensively studied, yet separately conducted for its brackish and the fresh water regimes, even though they are a tightly coupled aquatic system.
Oceanographic specialists covered estuary types (Chung et al. [1983]; Oh et al. . [1995]); Lee et al. [1999]), nutrient variations (Ki [1987]; Lee & Yang [1997]; Yang et al. [1999]; Jeong et al. [2005]), and siltation problems (Chough & Kim [1981]; Kim et al. [2006]). Concurrently, limnological studies have dealt with the phytoplankton community (Rim et al. [2000]; Shin & Cho [2000]) and environmental chemistry (Kim & Lim [1997]; Hwang et al. [2001]; Chung & Hwang [2002]; Lee et al. [2005]). Therefore, as a comparative summary of the previous investigations, this work compiles changes of aquatic conditions in the GREDS, focused on the physical, chemical, and biological changes of the estuary and lacustrine sides between pre-dam and post-dam.
2. GEOGRAPHIC SETTING
The Geum River Estuary, which is located in the southwestern part of the Korean Peninsula, exhibits typical characteristics of a well-mixed estuary. This characteristic is strongly related to the shallow depth of the estuary and the large tidal range at its mouth. The Geum River is one of the major rivers in Korea with the drainage area of approximately 100,000 km2 mostly composed of Precambrian metasedimentary rocks. This river drains through the Geum River Estuary to the Yellow Sea (Fig. 1). Its annual freshwater discharge is approximately 6 billion tons, mostly concentrated in the wet season, causing great seasonal variation in the river flow (Lee & Kim [1987]). Accordingly, annual sedimentation loads are estimated to be 5.6×106 tons year-1, also showing distinct seasonal variations (Chough & Kim [1981]).
The coastal zone shows a semi-diurnal macro-tidal regime with a mean tidal range of 4.3 m. The average spring and neap tidal ranges at the estuary are 5.7 m and 2.8 m, respectively, demonstrating a wide range of variation in the tidal energy input into the estuary. The spring-tidal saline water penetrates to about 60 km upstream, forming an extensive estuarine environment.
In 1994, KRC constructed a dam, the GREDS, to reserve freshwater approximately 20 km upstream from the mouth of the river, which resulted in an elongated funnel shaped reservoir that is 2 km wide, 40 km long, and 3-6 m deep.
3. PRECIPITATION
Generally, high precipitation in any watershed is assumed to cause a high flow-rate of rivers and a high water-level in a downstream reservoir. However, in reality, this is not always the case. For a reservoir located near any highly populated area, when a heavy rainstorm is expected, the sluice gate operator is apt to keep the water level of the reservoir down to its lowest level to avoid inundation of the surrounding farmland and housing areas. Such a manually generated low water-level of the reservoir probably keeps the actual water volume of the basin much smaller than usual, at least during the early stage of the wet seasons. Therefore, monthly-accumulated precipitation positively correlated with the flow rate of the Geum River nonetheless correlates negatively with the water level of the reservoir
3. PHYSICAL IMPACTS
3.1 Limited water mixing in the estuary
The water mixing regime is the most important factor in mass balancing and cycling of materials in an estuary (Knauss [1997]). After the dam building, such a mixing regime changed the circulation scheme of the estuary, consequently decreasing maximum tidal velocity by 65% from 3.5-3.8 knot to 1.5-2.2 knot during the spring tide period (Table 1).
Additionally, in situ observation showed an eminent residual landward flow at the bottom of the estuary during middle and high tide periods with low river discharge (Chung et al. [1983]; Lee et al. [1999]). Vertical stratification of water column with two opposite directions of residual currents; seaward flow at the surface and landward flow at the bottom layer were observed (Choi et al. [1995]); Kim et al. [2006]). Therefore, the dam not only physically transformed the lacustrine water into a freshwater regime but also changed the estuary to a partially-mixed type, which restricts active mixing of fresh and saline water in the estuary (Fig. 3).
4.2 Hydraulic residence time (HRT) in the lacustrine water
HRT is determined by the average length of time that water remains within the boundaries of an aquatic system, and it is a key parameter in controlling the biogeochemical behavior of aquatic ecosystems (Rueda et al. [2006]). HRT in the lacustrine water is defined as following a simple equation, such as T = V/R, where, V and R are the volume of the reservoir and the river discharge, respectively (Table 1). Unlike the estuary, the lacustrine side of the dam transformed to a fully fresh-water regime, and consequently, HRT depends on the volume of the lacustrine and the river discharge. Assuming a consistent water level of the reservoir, its HRT depends totally on the river discharge of the Geum River. Therefore, the frequent heavy rainstorms and manually controlled low water-level of the reservoir resulted prolonging the HRT by a factor of five to 11.6 days during the wet seasons; and HRT was prolonged even more greatly by a factor of 10 to 23.78 days during the dry season after the damming.
4.3 Estuarine HRT
Unlike the lacustrine HRT, estuarine HRT (Tr) is defined as the time required to replace all of the water with the tidal volume and river discharge (Table 1). The numerators, V, P, and T, stand for the low-tide volume, the tidal prism, and the tidal period of the estuary, respectively. This entire component of numerators represents the time-averaged sum of all of the water volume (capacity) in the estuary. The denominators, b and R, symbolize the return flow factor (set to 0.5 (USEPA [1985])) and the river discharge, respectively. These two components correspond to the actual time-averaged sum of all of the water volume (tidal flow and river discharge) in the estuary. Therefore, Tr (HRT) means the time required to replace all of the estuarine water with the tidal volume and river discharge. The actual difference in the HRT calculations between the estuary and the reservoir is their water composition, as there is only freshwater in the reservoir, and there is seawater plus freshwater in the estuary.
Since the dam was built, the maximum velocity of the tidal current has decreased, as did the tidal prism of the estuary (Table 1; also refer Kim et al. [2006]). The tidal prism, expressed as P, was reduced in both the numerators and denominators, but slightly less in the denominator due to the effects of the return factor, R. The estuarine HRT was not considerably different from the pre-dam situation, only prolonged by 22.1%. The HRTs increased on both the lacustrine and estuarine sides but to a much greater extent in the lacustrine water. Therefore, the physical consequences after the dam building, such as the extended HRT, the limited tidal current, and the freshwater regime, were much more noticeable in the reservoir than in the estuary.
5. DETEORATED WATER QUALITIES
5.1 Elevated nutrients concentrations
Since the dam was built, due to limited tidal currents in the estuary, the high concentrations of SS in the estuarine and lacustrine water subsided down to 41.7 mg L-1 and 21.9 mg L-1, respectively (Fig. 4). In addition, much more significant increase of PO43-, NO3-, and NH4+: have been found in lacustrine water (130%, 98%, and 193%) than estuarine water (200%, 168%, and 767%) (Table 1).
The lacustrine water showed less turbidity, containing half of the SS concentration of the estuarine water. Consequently, there is much less photo-inhibition of APP in the reservoir than in the estuary. Since the dam was built, the water quality on both sides of the dam have experienced elevated nutrient levels and water clarity, though the lacustrine water suffered from deteriorated water quality more seriously due to greatly escalated Chl-a and nutrients levels than the estuarine water (Jeong et al. [2010]). These concentrations were so high that this lacustrine water quality represented a hypertrophic state according to the OECD Water Quality Standards.
5.2 A bay-like character estuary
During the wet season, the increased river in-flow due to high precipitation predominant other physico-chemical processes in the estuary. However, the lacustrine effluents flow into the estuary sporadically through the sluice gate during the dry seasons. Due to these strong seasonality, the water quality of the estuary strongly depends on the fluvial input manifesting a conservative character for NO3-, NO2- and SiO2- throughout the year, though there is an additive pattern for PO43- and NH4+ during the wet seasons (Yang et al. [1999]). Additional processes affecting nutrients are desorption processes from SS and the destruction of organic materials, mainly from phytoplankton biomass contained in the lacustrine effluents. During the dry seasons, a low salinity area existed, ranging from 515 psu, within less than 1 km away from the sluice gate of the dam. Therefore, unlike the pre-dam period, the estuary maintained a bay-like character, especially during the dry seasons in the post-dam period.
6. PHYTOPLANKTON COMMUNITIES
6.1 Elevated autochthonous primary production (APP) in the reservoir
Based on another hyper-trophic estuary dam in Korea, the Nakdong Reservoir, autochthonous primary preproduction (APP) is usually under the strong influence of phytoplankton biomass, irradiance and nutrient availability (Jeong & Cho [2004]). Because phytoplankton biomass and nutrients were sufficient for APP, it was concluded that the lacustrine APP linearly correlated with total irradiance. Accordingly, the APP exceeded the production of allochthonous origin (Choi [1994]), and accounted for more than 50% of the organic load (Shin et al. [1996]).
We observed an almost identical situation in the GREDS. Over the last two decades, the Chl-a concentration has increased by more than one order of magnitude, and other APP-related parameters, such as DO, pH, and COD, have increased by a factor of two (Jeong et al. [2010]). After the dam building, nutrients and DIN/DIP concentrations increased by several folds, and their seasonal fluctuations became much greater than before. Therefore, APP has increased much more in the lacustrine water than in the estuarine water since the dam building.
6.2 Harmful algal bloom (HAB) in the reservoir
Before the dam building, the dominant phytoplankton species in the lacustrine water were benthic brackish diatoms, such as Navicula, Skeletonema, and Thalassiosira (Shim & Yang [1982]). At that time, strong tidal currents and murky water inhibited primary production, so that a very low autochthonous algal biomass and very low phytoplankton species diversity were present. However, after the dam building, the lacustrine side changed to a freshwater regime, and planktonic fresh water diatoms such as Stephanodiscus appeared to be dominant in winter, and the blue-green algae Microcystis became dominant in summer (Shin & Cho [2000], also refer Table 1).
Microcystis, a well-known HAB species, prefer high temperature and phosphate concentration with lentic water, instead, diatoms require optimum soluble reactive nutrient ration of 1 (Si:N) for active growth (Humborg et al. [1997]). Based on this suggested that frequency of diatom blooms over the last decades has decreased and dinoflagellates have become more important, especially during summer, due to the dam building. Still this hypothesis is hard to prove because of constantly changing nutrient concentration and other conditions involved in the GREDS, such as northern jetty, etc.
6.3 Phytoplankton communities in the estuary
Since the dam was built, autochthonous blooms of Mesodiniumrubrum with concentrations of 6,950-8,650 cells ml-1 have repeatedly appeared in the estuary (Yih et al. [2000]). This euryhaline species is known to compete with other coastal plankton, with a wider tolerance to salinity and less dynamic conditions in the post-dam period. Addition to this species, other dominant phytoplankton were euryhaline coastal diatoms such as Skeletonema costatum, Asterionella glacialis and Thalassiosira nordenskiodii, and freshwater algae such as Anabaena sp., Oscilatoria sp., Stephanodiscus hantzscii, Pediastrum boryanum and Cryptomonads, which probably simply passively flowed into the estuary with lacustrine effluent (Table 1).
7. SERIOUS SILTATION
7.1 Increased siltation rate in the reservoir
Siltation is a major problem in reservoirs and dams because it reduces water storage capacity, threatens their roles as water supplies, shortens their lifetime, and finally increases their maintenance cost. Every year, approximately 880 million tons of agricultural soil is deposited in American reservoirs (Biswas [1996]). Thus, siltation has posed a major problem for the economic life of dams around the world.
The water basin of the Geum River is one of the most intensively farmed agricultural areas in Korea, and conventional tillage practices in this watershed often result in excessive soil erosion, leading to increased turbidity in the Geum River flow, especially during the wet season, including the Asian monsoon period.
Recent soundings of the lacustrine bottom topography in this reservoir recorded approximately 3-7 m thick sediment that has accumulated at the bottom of the reservoir after the dam building (Lee et al. [2005]). This previous study also found mud layers at the surface, an alternating silty-sand and mud layer in the middle, and a sand layer at the bottom, to a depth of 7 m. Before the dam building, there were only sand layers because of the dynamic condition of the estuary; all of the mud and silty-sand layers accumulated afterwards. For instance, based on a simple calculation of an average sedimentation rate of 500 cm 20 years-1, and assuming this sedimentation rate maintains in the future, we obtained a sedimentation rate of 25 cm year-1. Thus, as the average depth of the reservoir is only 3 m, we calculate that it will be filled by siltation within 15 years. Even though this kind of calculation may be rough and possibly incorrect in some aspect, the calculated rate is high enough to attract our attention.
Before the dam building, 5.6×106 tons year-1 of sediment were transported to the estuary by the Geum River (Chough & Kim [1981]). However, this reduced to 2.94×106 tons year-1 after the dam building (Kim et al. [2006]). Thus, we could easily estimate an additional 1.83×106 m3 year-1 of sediment trapped in the reservoir. A previous study reported 0.56×106 m3 year-1 of sedimentation in the reservoir before the dam building (KRC [1996]), so annual sedimentation increased by 209% after the dam building (Table 1). Therefore, the GREDS traps a significant portion of the river-transported sediment that passes through this system. Due to such a rapid accrual of sediment, the reservoir will not be able to be used for its original purposes in the next decade (Lee et al. [2005]). Therefore, this serious siltation problem is threatening the original role of the GREDS.
7.2 Changed sedimentary condition in the estuary
Before dam building, the estuary showed characteristics of a typical macro-tidal estuary, with its tidal excursion reaching up to 60 km upstream from the mouth of the estuary (Fig. 1). At that time, an enormous volume (approximately 1.0×109 m3 day-1) of tidal floods and ebb currents swept over the entire channel of the estuary, and the HRT of the estuary was estimated to be 1.90 and 1.94 days for the wet and dry seasons, respectively (Table 1). However, the maximum tidal current velocity decreased by 65.5%, changing the estuary to a partially mixed type characterized by vertical stratification during the dry seasons after the dam building (Fig. 3). Under this calm condition, abundant particulate matter began to settle down to the bottom, and SS concentration in the water column decreased by 73.0% from 154.3 mg L-1 to 41.7 mg L-1 on average (Fig. 4). Thus, the sedimentation rate increased by 91%, from 3.5×106 m3 year-1 to 6.7×106 m3 year-1 (Table 1), with deposition as high as 32-66 cm year-1 in the estuary after the dam building (Kim et al. [2006]).
In addition to this increased sedimentation rate, the composition of sediment also changed. Because the reservoir efficiently traps relatively large grain particles before reaching the estuary (Lee et al. [2005]) and because of the less dynamic conditions due to the weak tidal action in the post-dam period, most bottom sediments are covered by mud deposits in the estuary (Kim et al. [2006]).
For the GREDS, the sediment in both the estuary and reservoir are accumulating so rapidly that the reservoir will be filled up in the next decade, and the estuary will no longer function as a harbor. Thus, due to such an extremely high sedimentation rate, the GREDS will not be usable for its original purposes in the next decade.
7.3 Accumulation of organic-rich sediment in the reservoir
The inflow of a large amount of total phosphorous (TP) to reservoirs results mainly from erosion of soil particles from steep slopes and disturbed ground during floods and monsoons (An & Park [2002]). Thus, TP is commonly associated with soils, while only a small percentage of the TP in freshwater is in the soluble forms of PO43- and its polymers. In the watershed, PO43- is readily immobilized in the soil, and even that from fertilizers soon becomes unavailable for plankton growth. Thus, in contrast to other nutrients, PO43- does not move easily with ground water to recharge surface streams (Horne & Goldman [1994]). More than 90% of TP eroded from continental rocks is carried to the sea through estuaries in an inert form, and total SS positively correlated with TP in Missouri reservoirs (Hoyer & Jones [1983]; Jones & Knowlton [1993]). Consequently, phosphorous, especially its particulate fraction, may have settled under tranquil hydraulic conditions, like those present the post-dam period, together with inorganic particulate materials, such as SS.
As improved water clarity with enhanced light penetration provides better condition for primary production, acceleration of APP was observed by previous investigators soon after impoundment of oxbow reservoirs (Cullum et al. [2006]). They reported that reduced sediment-laden run-off should reduce SS concentrations in the receiving water of reservoirs. Recently, frequent autochthonous algal blooms have produced abundant organic materials in lacustrine water, and significant parts of those settled down to the bottom of the reservoir (Shin & Cho [2000]). Consequently, the surface sediment of the reservoir is covered mostly by a thick mud layer containing rich organic content. According to the preceding investigators, the chemical properties of the sediment in the reservoir showed that organic carbon, TP, organic P, and ignition loss were 0.75-1.66%, 680-1,237 mg kg-1, 200-705 mg kg-1, and 3.0-10.9%, respectively (Kim and Lim [1997]; Hwang et al. [2001]; Chung and Hwang [2002]; Lee et al. [2005]). Moreover, they estimated the maximum releasing rate from sediment as 16.6 mg TP m-2 day-1, which would correspond to 35% of the mean TP concentration in the lacustrine water. Therefore, silty sediment with rich organic content exists at the surface of the lacustrine bottom sediment, due to the active APP in the reservoir.
7.4 Organic rich sediment and turbidity maximum zone (TMZ) in the estuary
Turbidity maximum zones (TMZ) exist in many estuaries of the world, being important with respect to the sedimentary processes of fine materials in estuaries (Allen et al. [1974]; Doxaran et al. [2009]) and to its evolution pattern in temporal and spatial contexts (Uncles et al. [2002]). For the macro-tidal estuarine environment before dam building, a TMZ existed in the middle of the estuary approximately 15-20 km from the mouth (Lee & Kim [1987]). As schematically illustrated (Fig. 5a; also refer Kim et al. [2006]), the TMZ was generated during the period of the spring tide, and it was concentrated at the middle of the estuary during the neap tide, while it was flushed out from the estuary during the high river discharge period. Therefore, the tidal current and fluvial regime was two key parameters causing the existence of the TMZ. In the central core of the TMZ, an SS concentration up to 1,000 mg L-1 was reported at the bottom layer during the spring tides (Lee & Kim [1987]), which moved back and forth with tidal excursions along the estuary and was flushed out of the estuary with high river discharge.
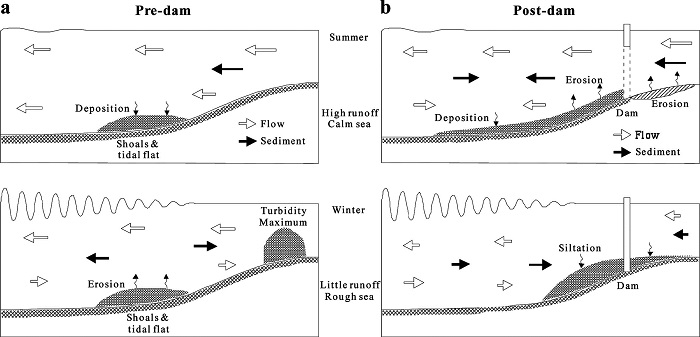
Schematized diagrams of seasonal sedimentation processes in the Geum River Estuary Dam System under (a) pre- and (b) post-dam conditions. The open arrow indicates residual flow and the filled net sediment transport. The magnitude of net flow and sediment flux is expressed implicitly by the length of the arrows. Erosion occurs during high river runoff in rainy summer and accumulation of sediment in dry winter at the adjacent location to the dam (Kim et al. [2006]). Publication permitted with the written consent from the Elsevier Publisher and “Estuarine, Coastal and Shelf Science”.
Unlike in the pre-dam period, the current tidal excursion is limited to only 15 km from the mouth of the estuary by the dam, and the maximum velocity of the tidal current has decreased by 65%, with SS dwindling down to one fifth of its pre-dam levels. The existence of a high SS concentration in the estuary would likely be the result of strong resuspension of sediment and/or intrusion of seawater carrying suspended sediment from coastal waters (Uncles et al. [2002]). In the GREDS, the strong tidal current has diminished, though fine particulate materials with rich organic content continue to flow into the estuary. The fine particles in the estuary originated from accelerated APP in the reservoir. Therefore, the sedimentary scheme of the estuary has changed, as illustrated in Fig. 5b (Kim et al. [2006]).
Even after dam building, fine particles and dissolved organic materials from the reservoir continue to flow into the estuary, produce flocculation in contact with saline water, and settle down near the sluice gate of the dam, where there is organic rich sediment. As a result, a high sedimentation rate up to 32 cm year-1 and rich organic content was observed in the estuary (Kim et al. [2006]).
The most significant characteristic of an estuary is the mixing of freshwater and seawater, which are different in their physical and chemical natures (Aston [1978]). The inflow of freshwater into the dense seawater in an estuary produces a vertical salinity gradient, and this vertical stratification controls the transport of water and SS within an estuary (Allen et al. [1980]). Because the Geum River Estuary showed a wide tidal range, the fluvial in-flow and tidal conditions seem to be responsible for the classification of the estuary. As the vertical salinity profiles showed particularly high stratification profiles ranging from 5-20 psu (Fig. 3), the estuarine mixing type with two-layer flow has become predominant, suggesting the influence of freshwater at the surface layer and saline water at the bottom. During the flood tide, landward transport prevailed in the estuary; in addition to this type of transport, secondary landward movement accompanied the returning flow with highly concentrated bottom sediment and coagulations of dissolved river-transported materials (Allen et al. [1980]). After dam building, the altered sedimentary scheme in the estuary, characterized by less dynamic conditions and a stratified water column, induced the high accumulation rate of sediment with rich organic contents.
8. FATE OF RIVER-TRANSPORTED MATERIALS
8.1 Trapping of river-transported materials in the reservoir
The Geum River Estuary was a typical estuary with dynamic physical conditions with macro-tidal amplitude of 7.5 m, a high concentration of suspended solids of frequently more than 150 mg L-1 (Lee & Yang [1997]), and low primary productivity due to high turbidity (Shim & Yang [1982]) before the dam building. At that time, extremely murky water from high SS content was an important inhibiting factor limiting autochthonous primary preproduction (APP), even though there were sufficient dissolved nutrients in water. Therefore, materials in the fluvial water, such as nutrients, pass through the estuary intact without being fully depleted by phytoplankton consumption. Because of this, the estuary was functioning almost as a simple passage for river transportation of materials to coastal waters.
Since the dam was built in 1994, two-thirds of the total estuary area have transformed from a brackish to a fresh-water regime, rapidly replacing salt water. Consequently, most of the SS in the lacustrine water settled down to the bottom, and eventually the water transparency improved (Fig. 4b). Correspondingly, calm water with high transparency, high nutrient concentrations and elongated HRT in the lacustrine water has provided an ideal condition for the growth of phytoplankton. Consequently, plentiful nutrients in clam water probably have a much greater opportunity of being consumed by APP. Finally, the lacustrine water after dam building transformed to a highly eutrophic water body with frequent autochthonous algal blooms and elevated nutrient concentrations (Jeong et al. [2010]). Eventually, the lacustrine water became extremely productive, and the active conversion of nutrients to organic materials occurred. During the last two decades, significant amounts of NH4+ and PO43- have been removed from the lacustrine water, while a corresponding quantity of Chl-a and organic materials have been generated in the lacustrine water. Therefore, this active transformation of dissolved nutrients into particulate organic materials in the reservoir has converted the role of the Geum River Estuary from a simple passage to an effective trap of river-transported materials.
8.2 Ecological consequences due to the excessive trapping of river-transported fine particles in the estuary: a potential hazard to coastal water
The estuary used to behave like a passage for river-transported dissolved nutrients because of its dynamic conditions with aggravated suspended solids and poor clarity. Such a condition is inhibitory to primary production, even with plenty of nutrients.
Estuaries, in nature, represent a pathway for materials from land to coastal waters and function as a filtering system. However, the artificial structures like the estuary dams disrupt their normal functions as estuaries.
After the dam building, only one-third of the total estuarine area has remained as an estuary. Strong tidal currents no longer exist, and SS has decreased to one fifth of its previous concentrations. Therefore, photo-inhibition of primary production has disappeared. In addition, the sedimentary scheme has changed. The TMZ has disappeared, and the stratification of the water column has produced a two-layer system with freshwater at the surface and saline water at the bottom. Moreover, an evident landward movement of saline water through the bottom layer has proved to be a favorable condition for the sedimentation of fine particles, especially coagulated materials formed from freshwater contacting saline water. Through this process, rich organic sediments have accumulated at very near to the sluice gate of the lacustrine region. Currently, even with only one-third of its original area, the estuary traps sediment and organic matter more efficiently than before. Therefore, the estuary is no longer functioning as a passage for river-transported materials, but rather like an efficient trap (Table 2). One of the active removal process of materials due to the damming, such as “missing silicate” hypothesis probably explain one of the reasons for the declines of coastal fisheries in the Black Sea (Humborg et al. [1997]).
9. CLOSING REMARKS
As a comparative summary of the previous investigations, this work compiles the disrupted aquatic conditions of the GREDS, focused on the similarities and differences between the estuarine and the lacustrine sides. Our discussion based on the review (Friedl & Wüest [2002]) regarding to aquatic disturbances of freshwater regimes by damming that impoundment of fresh water effects not only the hydrology of the ecosystem, but also its physical, chemical, and biological characteristic. Herein, we found such effects commonly appeared in the both lacustrine and the estuarine sides of the GREDS. However, some impacts such as elongated residence time, increased water clarity and elevated nutrients concentrations, siltation, and frequent outbreaks of harmful algal blooms are much more noticeable in the lacustrine side than in the estuarine side. Among them, harmful algal blooms are threatening proper usage of the freshwater, which requires urgent measures. Still, some disturbances in the estuarine side are not noticeable, yet the disturbance, such as excessive trapping of river-transported materials prior reaching to coastal water, is going to disrupt the geochemical balance of materials in the coastal ecosystem, which probably influences the coastal primary production and local fisheries in the future (Table 2). The disruptions of water quality such as high nutrients concentration and frequent bloom of blue-green algal are much more noticeable in the reservoir than in the estuary. However serious siltation problem has been reported from the estuary, which will possibly threaten the proper functioning of the natural Kunsan Estuarine System.
In the future, rather long-term environmental consequences, such as collapse of coastal fisheries and more frequent dinoflagellates bloom, would be expected in the coastal environment due to the excessive trapping of river-transported materials.
Acknowledgments
This research was a part of project “Saemangeum coastal system research for marine environmental conservation” and “Environment impact assessment and the related technologies on the freshwater intrusion to tidalflat: focused on the field applicable technologies with mesocosm” funded by Ministry of Land, Transport and Maritime Affairs, Korea.
References
-
G.P Allen, J.C Salomon, P Bsssoullet, Y Du Penhoat, C de Grandpré, Effects of tides on mixing and suspended sediment transport in macrotidal estuaries, Sediment. Geol, (1980), 26, p69-90.
[https://doi.org/10.1016/0037-0738(80)90006-8]
- G.P Allen, P Castaing, A Klingebiel, Suspended sediment transport and deposition in the Gironde estuary and adjacent shelf, Mem. Inst. Geol. Bassin Aquitaine, (1974), 7, p27-36.
-
K.G An, J.R Jones, Temporal and spatial patterns in salinity and suspended solids in a reservoir influenced by the Asian monsoon, Hydrobiologia, (2000), 436, p179-189.
[https://doi.org/10.1023/A:1026578117878]
-
K.G An, S.S Park, Indirect influence of the summer monsoon on chlorophyll-total phosphorus models in reservoirs: a case study, Ecol. Model, (2002), 152, p191-203.
[https://doi.org/10.1016/S0304-3800(02)00020-0]
- S.R Aston, Estuarine chemistry. In: Chemical Oceanography (eds J.P. Riley & R. Chester), Academic Press, London, (1978), p361-440.
-
R.M Baxter, Environmental effects of dams and impoundments, Annu. Rev. Ecol. Syst, (1977), 8, p255-283.
[https://doi.org/10.1146/annurev.es.08.110177.001351]
- A.K Biswas, Water Resources-Environmental Planning, Management and Development, McGraw-Hill, New York, (1996).
- J.S Choi, Organic Matter Budget and the Contribution of Autochthonous Organic Loading by Primary Productivity of Phytoplankton, MS thesis, Kangwon National University (in Korean with English abstract), (1994).
- S.K Chough, D.C Kim, Dispersal of fine grained sediments in the southeastern Yellow Sea: a steady-state model, J. Sediment. Res, (1981), 51, p721-728.
- J.Y Choi, H.Y Choi, M.S Seo, Physical and sedimentological changes in the Keum Estuary after the gate-close of Keum River weir, J. Korean Soc. Oceanogr, (1995), 30, p262-270, (in Korean with English abstract).
- J.Y Chung, J.H Lee, H.S An, Circulation dynamics of the Keum River Estuary I, Variability of the salinity boundary layer. J. Oceanol. Soc. Korea, (1983), 18, p142-148, (in Korean with English abstract).
- Y.S Chung, J.Y Hwang, Organics included in thesediments from Daecheong and Keum-River Estuary Reservoirs, J. Kor. Soc. Env. Anal, (2002), 5, p113-118, (in Korean with English abstract).
-
R.F Cullum, S.S Knight, C.M Cooper, S Smith, Combined effects of best management practices on water quality in oxbow lakes form agricultural watersheds, Soil Till. Res, (2006), 90, p212-221.
[https://doi.org/10.1016/j.still.2005.09.004]
-
D Doxaran, J.M Froidefond, P Castaing, M Babin, Dynamics of the turbidity maximum zone in a macrotidal estuary (the Gironde, France): Observations from field and MODIS satellite data, Estuarine, Coastal Shelf Sci, (2009), 81, p321-332.
[https://doi.org/10.1016/j.ecss.2008.11.013]
-
U Förstner, Sediment dynamics and pollutant mobility in rivers: An interdisciplinary approach, Lakes Reservoirs Res. Manage, (2004), 9, p25-40.
[https://doi.org/10.1111/j.1440-1770.2004.00231.x]
-
G Friedl, A Wüest, Disrupting biogeochemical cyclesconsequences of damming, Aquat. Sci, (2002), 64, p55-65.
[https://doi.org/10.1007/s00027-002-8054-0]
-
A.M Geraldes, M.J Boavida, Limnological comparison of new reservoir with one almost 40 years old which had been totally emptied and refilled, Lakes Reservoirs Res. Manage, (1999), 4, p15-22.
[https://doi.org/10.1046/j.1440-1770.1999.00075.x]
- P.H Gleick, The World’s Water 1998-1999, Island Press, Washington, D.C, (1999).
-
L Håkanson, Internal loading: A new solution to an old problem in aquatic sciences, Lakes Reservoirs Res. Manage, (2004), 9, p3-23.
[https://doi.org/10.1111/j.1440-1770.2004.00230.x]
- A.J Horne, C.R Goldman, Goldman, Limnology, 2nd ed, McGraw-Hill, Inc., New York, (1994).
-
M.V Hoyer, J.R Jones, Factors affecting the relation between phosphorus and chlorophyll a in Midwestern reservoirs, Can. J. Fish. Aquat. Sci, (1983), 40, p192-199.
[https://doi.org/10.1139/f83-029]
-
C Humborg, V Ittekkot, A Cociasu, B.V Bodungen, Effects of Danube River dam on Black Sea biogeochemistry and ecosystem structure, Nature, (1997), 386, p385-388.
[https://doi.org/10.1038/386385a0]
- J.Y Hwang, W.S Chung, Y.S Chung, Phosphorus and metal content in the sediments from Daecheong and Keum- River Estuary Reservoirs, J. Kor. Soc. Env. Anal, (2001), 4, p85-91, (in Korean with English abstract).
- S.I Jeon, K.J Cho, Primary productivity of phytoplankton in the shallow and hypertrophic River (Seonakdong River), Korean J. Limnol, (2004), 37, p57-63.
- Y.H Jeong, Y.T Kim, Y.Z Chae, C.W Rhee, K.R Ko, S.Y Kim, J.Y Jeong, J.S Yang, Analysis of long-term monitoring data from the Geum River Estuary, The Sea, J. Korean Soc. Oceanogr, (2005), 10, p139-144, (in Korean with English abstract).
- Y.H Jeong, J.S Yang, Variation of water qualities due to an estuary dam, (Inpress), (2013).
-
J.R Jones, M.F Knowlton, Limnology of Missouri reservoirs: an analysis of regional patterns, Lake and Reservoir Management, (1993), 8, p17-30.
[https://doi.org/10.1080/07438149309354455]
-
J.R Jones, M.F Knowlton, Suspended solids in Missori reservoirs in relation to catchment features and internal processes, Water Res, (2005), 39, p3629-3635.
[https://doi.org/10.1016/j.watres.2005.06.007]
- J.H Ki, The Study of Nutrients Cycling in the Keum River Estuary, MS thesis, Seoul National University (in Korean with English abstract), (1987).
- H.S Kim, Y.G Kim, J.S Yang, W Yih, Comparative population dynamics of photosynthetic ciliate Mesodinium rubrum (=Myrionecta rubra) in Gomso Bay and the Geum River Estuary, Korea, The Sea, J. Korean Soc. Oceanogr, (2004), 9, p164-172, (in Korean with English abstract).
- T.E Kim, B.S Lim, A study on characteristics of water pollutions releasing from sediments in Keum River Estuary Reservoir, J. KSWQ, (1997), 13, p245-253, (in Korean with English abstract).
-
T.I Kim, B.H Choi, S.W Lee, Hydrodynamics and sedimentation induced by large-scale coastal developments in the Keum River Estuary, Korea, Estuarine, Coastal Shelf Sci, (2006), 68, p515-528.
[https://doi.org/10.1016/j.ecss.2006.03.003]
- J.A Knauss, Introduction to Physical Oceanography, 2nd ed., Prentice-Hall, Inc., New Jersey, (1997).
- KRC, Hydrologic Survey Report on Geum River(II). Korean Rural Community Corporation, Korea (in Korean), (1996).
- KRC, The Saemangeum Dyke and the New Development Plan: Past, Present and Future. Korean Rural Community Corporation, Korea, (2009).
- C.B Lee, T.I Kim, Formation and evolution of turbidity maximum in the Keum Estuary, west coast of Korea, J. Oceanol. Soc. Korea, (187), 22, p105-118.
- S.D Lee, G.Y Lee, J.K Park, A study on the physicochemical characteristics of sediments in the estuary of Geumgang (River), J. Korean Environ. Admin, (2005), 11, p189-197, (in Korean with English abstract).
- S.H Lee, H.K Kwon, H.Y Choi, J.S Yang, J.Y Choi, Physical environment changes in the Keum River Estuary by the dyke gate operation II. Salinity structure and estuary type, The Sea, J. Korean Soc. Oceanogr, (1999), 4, p255-265, (in Korean with English abstract).
- Y.H Lee, J.S Yang, 500-days continuous observation of nutrients, chlorophyll, suspended solid and salinity in the Keum Estuary, Korea, The Sea, J. Korean Soc. Oceanogr, (1997), 2, p1-7, (in Korean with English abstract).
-
A Lourantou, J.-P Thomé, A Goffart, Water quality assessment of a recently refilled reservoir: The case of Bütgenbach Reservoir, Belgium, Lakes Reservoirs Res. Manage, (2007), 12, p261-274.
[https://doi.org/10.1111/j.1440-1770.2007.00345.x]
- P McCully, Silenced River: The Ecology and Politics of Large Dams, Zed Books, London, (1996).
- I.S Oh, T.K Na, C.B Lee, Tidal current and suspended sediment transport in the Keum Estuary, west coast of Korea, J. Korean Soc. Oceanogr, (1995), 30, p147-162.
- C.S Rim, J.K Shin, K.J Cho, The trend and assessment of water pollution from midstream to downstream of the Kum River, Korean J. Limnol, (2000), 35, p51-60, (in Korean with English abstract).
-
D.M Rosenberg, F Berkes, R.A Bodaly, R.E Hecky, C.A Kelly, J.W.M Rudd, Large-scale impacts of hydroelectric development, Environ. Rev, (1997), 5, p27-54.
[https://doi.org/10.1139/a97-001]
-
F Rueda, E Moreno-Ostos, J Armengol, The residence time of river water in reservoirs, Ecol. Model, (2006), 191, p260-274.
[https://doi.org/10.1016/j.ecolmodel.2005.04.030]
- T Sawaragi, Current shore protection works in Japan, J. Coastal Res, (1988), 4, p531-541.
- J.K Shin, K.J Cho, Seasonal dynamics and pollution status of the water quality in the Kum River Reservoir, Korean J. Limnol, (2000), 33, p251-259, (in Korean with English abstract).
- S.K Shin, C.K Park, S.W Lee, The relationship between chlorophyll-a and BOD in the Nakdong River, J. KSWQ, (1996), 12, p369-375, (in Korean with English abstract).
- J.H Shim, J.S Yang, The community structure and distribution of phytoplankton of the Kum River Estuary, Estuary. J. Oceanol. Soc. Korea, (1982), 17, p1-11.
-
D.M Søballe, B.L Kimmel, A large-scale comparison of factors influencing phytoplankton abundance in rivers, lakes, and impoundments, Ecology, (1987), 68, p1943-1954.
[https://doi.org/10.2307/1939885]
-
S Tsujimura, Water management of Lake Yogo targeting internal phosphorus loading, Lakes Reservoirs Res. Manage, (2004), 9, p171-179.
[https://doi.org/10.1111/j.1440-1770.2004.00249.x]
-
R.J Uncles, J.A Stephens, R.E Smith, The dependence of estuarine turbidity on tidal intrusion length, tidal range and residence time, Cont. Shelf Res, (2002), 22, p1835-1856.
[https://doi.org/10.1016/S0278-4343(02)00041-9]
- USEPA, Coastal Marinas Assessment Handbook. United States Protection Agency, Region IV, Atlanta, Georgia, (1985).
-
G.A Weyhenmeyer, L Håkanson, M Meili, A validated model for daily variations in the flux, origin, and distribution of settling particles within lakes, Limnol. Oceanogr, (1997), 42, p1517-1529.
[https://doi.org/10.4319/lo.1997.42.7.1517]
- J.S Yang, J.Y Jeong, J.Y Heo, S.H Lee, J.Y Choi, Chemical mass balance of materials in the Keum River Estuary 1. Seasonal distribution of nutrients, The Sea, J. Korean Soc. Oceanogr, (1999), 4, p71-79, (in Korean with English abstract).
- W Yih, K.A Soung, H.S Kim, Y.M Park, Toward the establishment of routine laboratory culture of Mesodinium rubrum, a phototrophic marine ciliate, Bulletin of Coastal Research, Kunsan National University, (2000), 12, p61-69.